Sections
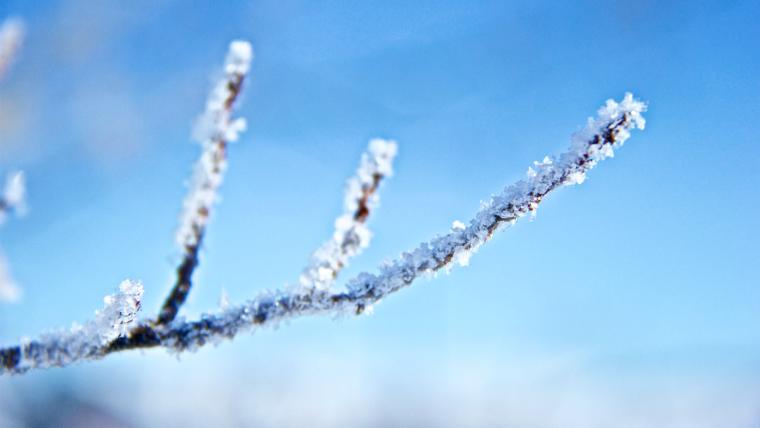
Trees damaged by late season frosts can see negative impacts for years
Fri, May 09, 2025
Many trees in the Northern Hemisphere are leafing out earlier as the climate warms. But with leaves emerging earlier in the season, there is an increased risk of damage from a late spring frost. Researchers used experimental and observational data – including thousands of records from Nature’s Notebook – to evaluate the effects of late spring frosts on hundreds of tree species. The results indicated that late spring frosts have both short-term and long-term effects on tree growth and phenology. In the short-term, late spring frosts damage young leaves and limit tree growth that year. But more notably, late spring frost events also limit the amount of resources a tree can store that year, which results in delayed leaf emergence and reduced productivity the next calendar year. These results illustrate that the effects of extreme climate events on plant phenology and productivity may extend months, or years, into the future. The results may also help scientists generate more accurate predictions of how forests will respond to future climate change.
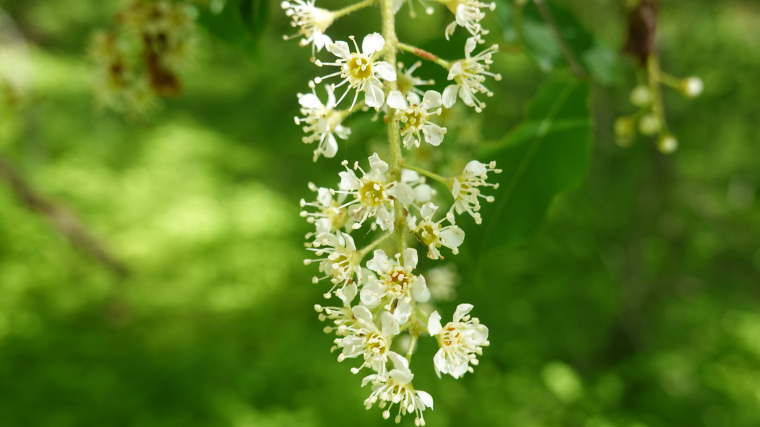
A more complete picture of the timing of spring
Thu, Mar 13, 2025
How early is spring this year and how does it compare to years past? We can answer this question using observations of plants and identifying the weather conditions that are needed for plants to leaf out and flower. For many years, the USA-NPN has shared maps, called the Spring Leaf Index and Spring Bloom Index, that show when weather conditions associated with events that signal the start of spring reached. However, an early start to spring does not necessarily mean that subsequent springtime events will also occur early. So, a team of researchers used thousands of observations submitted to Nature’s Notebook to identify conditions associated with events that occur later in the spring. And soon, maps for a Late Bloom Index, that depict the timing of activity in the latest-blooming plants will be added to the USA-NPN Status of Spring website. This new index, combined with the usual Spring Leaf and Spring Bloom Indices, will give us better understanding of how spring is progressing each year.
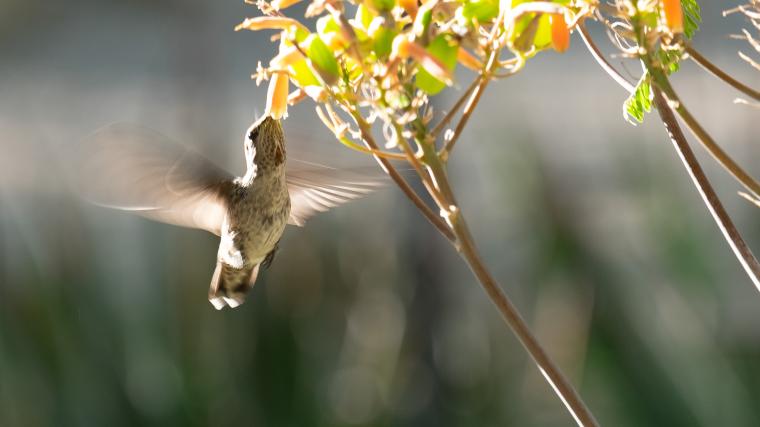
Are plant and animal species responding differently to climate change?
Tue, Jan 14, 2025
The advance in the timing of spring events is well documented in scientific research. However, changes have not been the same for all species and can even differ among populations of the same species in distinct locations. A team of researchers compiled an extensive dataset of phenology observations from the published literature and from phenology networks including USA-NPN. They estimated whether the average date that a life cycle stage occurs has shifted since 1980, assessed the extent to which those shifts were driven by changes in temperature or precipitation, and explored whether climate change was increasing the likelihood of phenological mismatches for species that occur in the same location. The authors found that for plants, spring and summer events like leaf out, flowering, and fruiting are occurring earlier than they did 40 years ago, with later stages like flowering and fruit ripening advancing faster than earlier stages. Climate had much weaker effects on the timing of animal activity, which has implications for mismatches in the timing of plants and animals that rely on synchronization of their life cycle stages.